Siberian Husky Genetics
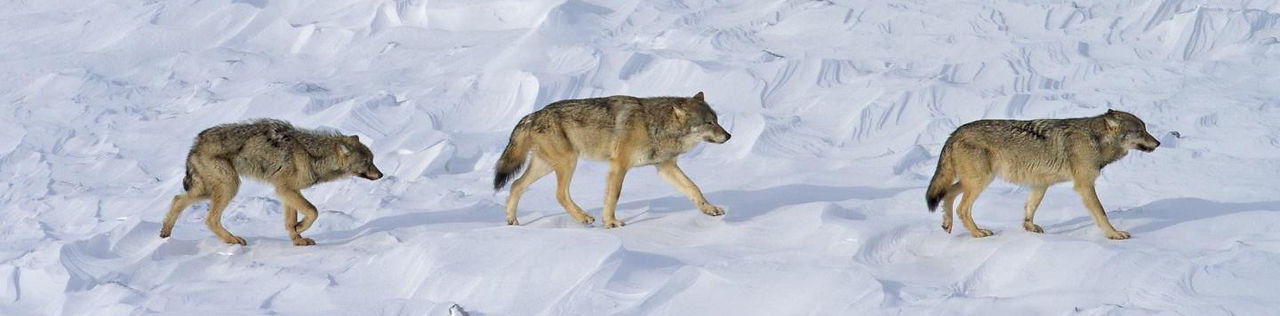
A 2015 photograph captures the last remaining male-female pair of Isle Royale wolves alongside one of their offspring (third in line, on the left), which exhibited visible deformities. The pup’s twisted tail and hunched posture suggested developmental abnormalities, and it is believed to have died before reaching nine months of age. These wolves were the final island-born members of the Isle Royale population.
Credit: Rolf O. Peterson, Michigan Technological University
Genetic Bottlenecks and Broken Defenses: The Immunological Cost of Inbreeding across the Animal Kingdom
By: Tracy Smith, PhD
Introduction
Inbreeding, the mating of closely related individuals, reshapes the genetic landscape of a population by increasing homozygosity and reducing genetic diversity. While this process can sometimes preserve desirable traits, it comes at a significant cost—heightened expression of harmful recessive alleles and a weakened ability to adapt to environmental challenges. One of the most critical consequences of inbreeding is inbreeding depression, a decline in key fitness traits such as survival, reproductive success, and disease resistance.
A particularly alarming effect of inbreeding is its impact on the immune system. With a narrower genetic repertoire, inbred individuals may struggle to recognize and combat a diverse array of pathogens, making them more susceptible to infections. A diminished immune gene pool can also increase the risk of autoimmune disorders, as improper immune regulation may lead to the body attacking its own tissues.
This review explores the genetic underpinnings of inbreeding’s effects on immunity, the evolutionary forces shaping these outcomes, and the real-world consequences for both animals and humans. By drawing from animal studies, conservation genetics, and human case studies, here I highlight the link between inbreeding and immune dysfunction, providing insight into why genetic diversity is essential for long-term health and survival.
Inbreeding and Immune Function: Genetic Mechanisms and Consequences
Inbreeding compromises immune function through two primary genetic mechanisms: loss of heterozygosity and expression of deleterious recessive alleles. Inbred individuals have reduced genetic variation at key immune-related loci, particularly in the Major Histocompatibility Complex (MHC), which plays a crucial role in pathogen recognition. In a healthy, outbred population, diverse MHC alleles enable the immune system to detect and respond to a wide range of infections. However, inbred populations often share the same MHC variants, limiting immune repertoire and increasing disease susceptibility (Lyons et al. 2009).
This phenomenon is evident in several highly inbred carnivore populations. Cheetahs, Florida panthers, and Isle Royale wolves all experienced severe bottlenecks, resulting in extreme genetic uniformity and raising concerns that a single virus could devastate an entire population.
Case Studies of Inbreeding and Disease Susceptibility

Cheetahs
Modern cheetahs, having passed through at least two major bottlenecks, are so genetically similar that even unrelated individuals accept skin grafts from each other—a clear indicator of MHC homogeneity. This lack of diversity has led to extraordinary disease vulnerability in captivity. In the 1980s, an outbreak of feline infectious peritonitis (FIP) at an Oregon wildlife park infected every cheetah in the population. The virus had a 100% seroconversion rate, and 90% of the cheetahs developed clinical disease, resulting in the deaths of 60% of the population—the most extreme coronavirus outbreak recorded in any species. Scientists concluded that the cheetahs’ genetic uniformity, particularly at immune-related genes, contributed to this catastrophic mortality. In a genetically diverse species, more individuals would have had natural resistance, preventing such a widespread outbreak.
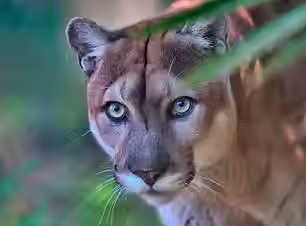
Florida Panthers
After a severe genetic bottleneck in the 20th century, Florida panthers became highly inbred, sharing similar immune weaknesses. Inbreeding depression manifested in physical abnormalities, including heart defects, undescended testicles, and weakened immune responses, leading to heavy parasite loads and poor disease resistance. In the early 2000s, five panthers died from feline leukemia virus (FeLV)—a disease previously unrecorded in the population but introduced by a domestic cat. Given the 100% mortality rate in infected panthers, conservationists launched an emergency vaccination campaign and enhanced disease surveillance to curb further spread. Concurrently, a genetic rescue program introducing Texas cougars helped restore genetic diversity, improving disease resistance and overall fitness in the panther population.

Isle Royale Wolves
Originally founded by just 2–3 wolves, the population peaked at 50 wolves in the 1980s but remained genetically stagnant due to the lack of new gene flow. By the 2000s, genome analysis revealed extensive runs of homozygosity, meaning individuals had inherited identical DNA segments from both parents. As a result, Isle Royale wolves exhibited 11–42% lower genome-wide heterozygosity compared to nearby mainland wolves (Robinson et al. 2019).
The consequences were severe. Many wolves developed spinal deformities (malformed thoracic and lumbar vertebrae), syndactyly (fused toes), cataracts, and other congenital anomalies—hallmarks of recessive mutations becoming homozygous. Survival and reproduction declined drastically (Hedrick et al. 2014), and by 2018, only two closely related wolves remained, a father-daughter pair that were also half-siblings (Robinson et al. 2019). The population had not produced viable pups since 2014, effectively making it nonviable (Peterson et al. 2017). Recognizing this crisis, wildlife managers initiated a genetic rescue in 2018, translocating wolves from the mainland to restore genetic diversity.

Fifty-eight percent of the severely inbred wolves from Isle Royale had congenital malformations in the lumbosacral region of the vertebral column, also known as transitional vertebrate.
Isle Royale’s wolves also faced devastating disease outbreaks, with their inbred status likely exacerbating their susceptibility. In 1980, a canine parvovirus (CPV2) outbreak reduced the population from ~50 wolves to only 12–14 (Robinson et al. 2019). Pup mortality was especially high, as young wolves are particularly vulnerable once maternal antibodies wane. Unlike larger, outbred wolf populations on the mainland, which rebounded after CPV outbreaks in the 1970s and 80s, the Isle Royale wolves remained at low numbers for over a decade. A brief genetic boost came in 1997, when a single migrant wolf (M93) arrived, increasing survival and reproduction. However, as his descendants interbred, inbreeding rose again, and the wolves’ health deteriorated. The pattern was clear: disease hits inbred populations harder, and recovery is slower, further driving the population’s decline.
Emerging research also suggests that low genetic diversity and inbreeding may increase cancer susceptibility through multiple mechanisms, both directly by increasing homozygosity of cancer-causing mutations and indirectly by impairing immune defenses, making individuals more vulnerable to cancer-causing pathogens (Ujvari et al., 2018). Inbred populations, whether in wildlife, domestic animals, or humans, often exhibit compromised immune function, reducing their ability to detect and eliminate cancerous cells or fend off oncogenic viruses and other infectious agents linked to tumor development.
One of the most compelling models for studying these effects is the domestic dog, where different breeds—each essentially a closed gene pool—exhibit vastly different cancer predispositions (Dobson 2013). Breeds which also happen to have higher inbreeding coefficients, such as Bernese Mountain Dogs, Boxers, and Golden Retrievers, are disproportionately affected by lymphomas, mast cell tumors, and osteosarcomas. This pattern underscores how restricted genetic variation can fix harmful alleles within a population, leading to an increased likelihood of hereditary cancers.
In both humans and other animals, immune suppression resulting from inbreeding is thought to further exacerbate cancer risk by increasing susceptibility to infectious agents known to drive tumor formation (e.g., human papillomavirus, Epstein-Barr virus, and Helicobacter pylori in humans; Tasmanian devil facial tumor disease in wildlife, canine transmissible venereal tumor in dogs). Given the far-reaching implications, this issue is of growing concern in conservation biology, epidemiology, and genetic research. Understanding the relationship between inbreeding, immune suppression, and cancer susceptibility is essential for developing effective conservation strategies, improving disease management, and refining genetic risk assessments for both endangered species and domesticated animals.
Evolutionary Perspectives on Inbreeding and Immune Resilience
From an evolutionary perspective, the detrimental effects of inbreeding on immunity help explain why most species have evolved mechanisms to avoid mating with close relatives. Inbreeding reduces offspring fitness, particularly by compromising their immune competence. Many animals have developed mate selection strategies that favor genetic dissimilarity, ensuring greater immune diversity in their offspring. For example, some mammals and fish can detect MHC differences through scent, preferring mates with more diverse MHC alleles to enhance their offspring’s immune defenses (Yamazaki et al. 2007). This strategy broadens the range of pathogens their immune system can recognize, increasing their resistance to disease. Similar mate preferences have been observed in humans (Winternitz et al. 2017), and throughout history, many cultures have established taboos against close-kin marriages, likely as an adaptive response to the harmful effects of inbreeding, including increased disease susceptibility.
Beyond mate choice, pathogens themselves drive the need for genetic diversity in host populations, a concept central to the Red Queen Hypothesis of host-parasite coevolution. As parasites and pathogens continually evolve, host populations must keep pace by shuffling genes through outbreeding, which produces novel immune defenses. Inbreeding disrupts this process by reducing genetic variability, making it easier for parasites to exploit genetically uniform hosts. Studies in wild populations support this idea: parasite-mediated selection tends to favor hosts with higher heterozygosity, while inbred populations, with fewer immune gene variants, are at greater risk of being overwhelmed by disease (Reid et al. 2003). Over generations, this lack of flexibility in responding to emerging infections can lead to reduced survival—a significant evolutionary cost. In extreme cases, epidemics can wipe out entire inbred populations because no individuals possess the genetic resistance needed to survive. This heightened disease vulnerability creates a strong selective pressure against inbreeding over evolutionary timescales.
While natural selection generally discourages inbreeding, it can be unavoidable in small or isolated populations. In such cases, some purging of the most harmful recessive alleles may occur—lineages carrying severe immune defects may not survive—but this process is incomplete and does not restore lost genetic diversity (Lacy 1997). Unlike mutations that cause immediate lethality, many deleterious immune-related mutations persist at low frequencies, accumulating over generations and weakening the population’s overall disease resistance. The consistency of inbreeding depression in immunity across species underscores the fundamental evolutionary advantage of maintaining genetic diversity for long-term immune resilience. Populations thrive when individuals breed outside their immediate family, maximizing genetic variety—especially at immune-related loci essential for fighting disease.
Health Consequences of Inbreeding on the Immune System
Increased Susceptibility to Infections
One of the most immediate consequences of inbreeding is a weakened immune response, leading to greater vulnerability to infectious diseases. Inbred individuals often struggle to mount effective defenses against pathogens, resulting in higher infection rates and more severe outcomes. Studies in wildlife and livestock consistently demonstrate that inbred animals harbor higher pathogen loads and reduced immune function. In Soay sheep and endangered gazelles, inbreeding correlated with higher parasite burdens and lower survival (Coltman et al., 1999; Cassinello et al., 2001). Similarly, inbred house mice exhibited weakened immune responses and greater mortality when experimentally exposed to pathogens (Meagher, Penn & Potts, 2000). In marine mammals, striped dolphins and harbor seals with lower genetic diversity suffered higher lung nematode burdens, reinforcing the link between homozygosity and immune suppression (Hoffman et al., 2014). Inbred European badgers suffered more severe bovine tuberculosis infections, further confirming that inbreeding undermines immune resilience across diverse mammalian species (Benton et al., 2018).
In domestic animals, a study of 50,000 dogs revealed that breeds with high inbreeding levels required significantly more veterinary care. Dogs with a genomic COI (coefficient of inbreeding) above 25% had a 1.29 times higher risk of veterinary visits compared to mixed-breed dogs, while low-inbreeding breeds had a nearly neutral risk factor of 1.01 (Bannasch et al. 2021). Although not all veterinary visits were infection-related, these findings highlight a clear trend: genetically diverse individuals are better equipped to resist infections and immune-related disorders, whereas inbred populations with reduced genetic variation are far more susceptible to disease and health issues.
Higher Risk of Primary Immunodeficiencies
A direct and severe consequence of inbreeding is the increased occurrence of rare immunodeficiency disorders. When parents are closely related, the likelihood of inheriting two copies of a recessive mutation rises significantly. If that mutation impairs a key immune function, such as T-cell development or antibody production, the result can be severe immunodeficiency from birth.
Clinical data from high-consanguinity populations illustrate this clearly. In regions where 20–50% of marriages occur between relatives (such as parts of the Middle East, South Asia, and North Africa), doctors report disproportionately high numbers of children with severe immunodeficiencies, including severe combined immunodeficiency (SCID) and chronic granulomatous disease. Many of these children would not have been affected if their parents were unrelated. A review of primary immunodeficiencies found that patients born to consanguineous parents experience significantly higher morbidity and mortality than those from genetically diverse backgrounds (Al-Herz et al. 2014).
This is because recessive immune defects, which are extremely rare in the general population, become much more common when the gene pool is restricted. The same pattern has been observed in small, isolated human groups, such as island communities and remote tribes, where limited genetic variation has been linked to weaker vaccine responses and higher prevalence of specific immune disorders.
Reduced Pathogen Resistance and Higher Outbreak Risk
At the population level, inbreeding compromises overall disease resistance, increasing the risk of large-scale outbreaks. When too many individuals share the same immune gene variants, a single virulent pathogen can spread rapidly, infecting nearly everyone. In contrast, a genetically diverse population contains individuals with different immune alleles, ensuring that some members will resist infection and break the chain of transmission. This concern is not just theoretical—it poses a serious threat to endangered species that have undergone genetic bottlenecks. Inbred populations of livestock and captive animals have also shown higher rates of disease outbreaks, often requiring extensive management to prevent collapse. Ultimately, reduced pathogen resistance due to inbreeding is a community-wide issue, compounding the threat of extinction for endangered species and creating public health and veterinary challenges.
Increased Autoimmune Disorders and Immune Dysregulation
Inbreeding does not just increase vulnerability to infections—it can also contribute to autoimmune diseases and immune dysregulation. Normally, genetic variation helps maintain a balance between immune activation and self-tolerance, but in inbred populations, harmful allele combinations can become concentrated, disrupting this equilibrium.
In humans, research on inbreeding and autoimmunity is still emerging, but evidence suggests that consanguineous populations (those with high rates of close-kin marriage) have higher frequencies of autoimmune disorders, including diabetes and heart disease. This could be linked to limited HLA (human MHC) diversity or the accumulation of specific immune risk alleles (Bener et al. 2017; Temaj et al. 2022).
In animals, the impact of inbreeding on autoimmune diseases is more clearly documented. Purebred dogs serve as a model, as each breed is essentially a closed gene pool derived from a small number of founders. Certain breeds suffer from multiple autoimmune conditions at far higher rates than mixed-breed dogs. For instance, Standard Poodles have an elevated risk of autoimmune hemolytic anemia, thyroiditis, and Addison’s disease, a likely consequence of intensive inbreeding for specific physical traits (Pedersen et al. 2015a).
A genetic study of poodles with Addison’s disease and sebaceous adenitis found that these conditions were concentrated in the most inbred lines, whereas dogs from more genetically diverse backgrounds were largely unaffected. No single gene was responsible; rather, genome-wide homozygosity and reduced immune gene diversity correlated with disease expression. A similar pattern was observed in Italian Greyhounds, where inbred individuals exhibited significantly higher rates of autoimmune disorders (Pedersen et al. 2015b). These findings suggest that an immune system shaped by a narrow genetic repertoire may struggle to properly regulate itself, increasing the likelihood of self-reactivity and chronic inflammation. In essence, inbreeding not only weakens pathogen resistance but can also disrupt immune balance, making affected individuals prone to autoimmune dysfunction.
Conclusion
The impact of inbreeding on the immune system is profound and well-documented across species. The primary genetic mechanisms—loss of immune gene diversity and expression of harmful recessive alleles—lead to measurable declines in immune competence. Evolution has consistently favored genetic mixing, as it enhances immune resilience and adaptability, whereas inbreeding weakens these defenses, leaving populations more vulnerable to infections, immune dysregulation, and long-term health decline.
A broad body of evidence, spanning controlled animal studies, natural population observations, and human epidemiological research, presents a clear and consistent pattern: inbreeding increases susceptibility to infections, raises the risk of immune disorders, and reduces overall health and survival. In small or isolated populations, where inbreeding accumulates over generations, the effects can be particularly severe. Without genetic diversity, populations lack the immunological flexibility to combat emerging infectious diseases, making them more susceptible to outbreaks that could threaten their survival. In extreme cases, entire inbred populations have been devastated by single pathogens, as seen in captive cheetahs with feline infectious peritonitis (FIP) and Isle Royale wolves during canine parvovirus outbreaks.
Conversely, preserving or restoring genetic diversity—whether through outbreeding, conservation-driven genetic rescue, or informed breeding strategies—can bolster immune function and improve population health. For endangered species like the Florida panther, this understanding has been critical to survival, guiding successful genetic rescue efforts that have revitalized the population and reduced disease susceptibility. Likewise, in human populations, growing awareness of the link between consanguinity and immune-related health risks is fostering better education, genetic counseling, and preventive healthcare strategies in communities where inbreeding remains culturally common.
Beyond individual and population health, the broader evolutionary implications of inbreeding’s impact on immunity highlight the role of host-pathogen coevolution. In a world where viruses, bacteria, and parasites constantly evolve, maintaining genetic diversity is not just beneficial but essential for long-term species survival. Populations with greater genetic variation are better equipped to withstand novel disease pressures, ensuring their resilience across generations. Ultimately, the connection between genetic diversity and immune function reinforces a fundamental biological principle: genetic variation is a critical asset for the survival of any species, shaping its ability to endure the ongoing evolutionary arms race with infectious diseases.
References
-
Acevedo-Whitehouse, K. & Cunningham, A. (2006). Is MHC enough for understanding wildlife immunogenetics? Trends in Ecology & Evolution, 21(8): 433–438.
-
Al-Herz, W. et al. (2014). Consanguinity and primary immunodeficiencies. Hum. Hered., 77(1-4): 138–143.
-
Bannasch, D., Famula, T., Donner, J., Anderson, H., Honkanen, L., Batcher, K., Safra, N., Thomasy, S. & Rebhun, R. (2021). The effect of inbreeding, body size, and morphology on health in dog breeds. Canine Medicine and Genetics, 8, pp.1-9.
-
Bener, A. & Mohammad, R.R. (2017). Global distribution of consanguinity and their impact on complex diseases: Genetic disorders from an endogamous population. Egyptian Journal of Medical Human Genetics, 18(4), pp.315-320.
-
Benton, C.H. et al. (2018). Inbreeding intensifies sex- and age-dependent disease in a wild mammal. Journal of Animal Ecology, 87(6): 1500–1511.
-
Calleri, D. V. et al. (2006). Inbreeding and disease resistance in a social insect. Proc. R. Soc. B, 273: 2633–2640.
-
Cassinello, J. et al. (2001). Relationship between coefficient of inbreeding and parasite burden in endangered gazelles. Conservation Biology, 15(4): 1171–1174.
-
Coltman, D.W. et al. (1999). Parasite-mediated selection against inbred Soay sheep in a free-living, island population. Evolution, 53(4): 1259–1267.
-
DeCandia, A.L., Schrom, E.C., Brandell, E.E., Stahler, D.R. & Vonholdt, B.M. (2021). Sarcoptic mange severity is associated with reduced genomic variation and evidence of selection in Yellowstone National Park wolves (Canis lupus). Evolutionary Applications, 14(2), pp.429-445.
-
Dobson, J.M., 2013. Breed‐predispositions to cancer in pedigree dogs. International Scholarly Research Notices, 2013(1), p.941275.
-
Florida Fish and Wildlife Conservation Commission. Florida Panther Genetics – Inbreeding Problems.
-
Foster, H.L., Small, J.D. & Fox, J.G. eds. (2014). The mouse in biomedical research: Normative biology, immunology, and husbandry. Academic Press.
-
Hedrick, P. W., Peterson, R. O., Vucetich, L. M., Adams, J. R. & Vucetich, J. A. (2014). Genetic rescue in Isle Royale wolves: Genetic analysis and the collapse of the population. Conserv. Genet., 15, 1111–1121.
-
Hoffman, J.I. et al. (2014). High-throughput sequencing reveals inbreeding depression in a natural population. PNAS, 111(13): 5130–5135. DOI: 10.1073/pnas.1318945111
-
Lacy, R.C. (1997). Importance of genetic variation to the viability of mammalian populations. Journal of Mammalogy, 78(2), pp.320-335.
-
Lyons, E. et al. (2009). Consanguinity and susceptibility to infectious diseases in humans. Biology Letters, 5(4): 574–576.
-
McCarthy, C. (2005). How does inbreeding affect the immune system of animals? The Tech Interactive (Ask a Geneticist).
-
Meagher, S., Penn, D. & Potts, W. (2000). Male–male competition magnifies inbreeding depression in wild house mice. Nature, 406: 69–72.
-
Miglior, F. et al. (1995). Nonadditive genetic effects and inbreeding depression for somatic cell counts of Holstein cattle. J. Dairy Science, 78(5): 1168–1173.
-
Pedersen, N. C. et al. (2015). Inbreeding effects on autoimmune disease in Standard Poodles. Canine Genetics and Epidemiology, 2: 14.
-
Pedersen, N.C., Liu, H., Leonard, A. & Griffioen, L. (2015). A search for genetic diversity among Italian Greyhounds from Continental Europe and the USA and the effect of inbreeding on susceptibility to autoimmune disease. Canine Genetics and Epidemiology, 2, pp.1-16.
-
Peterson, R. O. & Vucetich, J. A. (2017). Ecological Studies of Wolves on Isle Royale (Annual Report 2016-2017, Michigan Technological University).
-
Radwan, J., Biedrzycka, A. & Babik, W. (2010). Does reduced MHC diversity decrease viability of vertebrate populations? Biological Conservation, 143(3), pp.537-544.
-
Reid, J. M. et al. (2003). Inbreeding depresses immune response in song sparrows. Proc. R. Soc. B, 270(1529): 2157–2163.
-
Robinson, J.A., Räikkönen, J., Vucetich, L.M., Vucetich, J.A., Peterson, R.O., Lohmueller, K.E. & Wayne, R.K. (2019). Genomic signatures of extensive inbreeding in Isle Royale wolves, a population on the threshold of extinction. Science Advances, 5(5), p.eaau0757.
-
Ross-Gillespie, A., O'Riain, M.J. & Keller, L.F. (2007). Viral epizootic reveals inbreeding depression in a habitually inbreeding mammal. Evolution, 61(9), pp.2268-2273.
-
Temaj, G., Nuhii, N. & Sayer, J.A. (2022). The impact of consanguinity on human health and disease with an emphasis on rare diseases. Journal of Rare Diseases, 1(1), p.2.
-
Thorne, E.T. & Williams, E.S. (1988). Disease and endangered species: The black-footed ferret as a recent example. Conserv. Biol., 2: 66–74.
-
Ujvari, B., Klaassen, M., Raven, N., Russell, T., Vittecoq, M., Hamede, R., Thomas, F. and Madsen, T., (2018). Genetic diversity, inbreeding and cancer. Proceedings of the Royal Society B: Biological Sciences, 285(1875), p.20172589.
-
van de Kerk, M., Onorato, D.P. & Oli, M.K. (2015). The Florida Panther: Past, Present, and Future.
-
Winternitz, J., Abbate, J.L., Huchard, E., Havlíček, J. & Garamszegi, L. (2017). Patterns of MHC‐dependent mate selection in humans and nonhuman primates: A meta‐analysis. Molecular Ecology, 26(2), pp.668-688.
-
Yamazaki, K. & Beauchamp, G.K. (2007). Genetic basis for MHC‐dependent mate choice. Advances in Genetics, 59, pp.129-145.